Author: Ronen Shekel
Building large-scale quantum networks hinges on connecting different quantum systems, but these often operate at distinct optical wavelengths. Nitrogen-vacancy centers in diamond, for instance, are excellent candidates for quantum network nodes, emitting single photons at 637 nm [1].
However, transmitting these photons efficiently over long distances through standard optical fibers is problematic, as 637 nm light suffers significant loss. Conversion to the low-loss telecommunication bands is therefore essential.
Quantum frequency conversion (QFC) provides the solution, coherently shifting the wavelength of quantum light while preserving its crucial quantum properties, like entanglement or squeezing. Despite progress, achieving efficient QFC without introducing significant noise has remained a major challenge [2].
Even small amounts of noise can degrade or destroy the fragile quantum states, severely limiting quantum communication and networking capabilities.
A promising new platform, recently demonstrated by Mann et al. [3] using a crystal supplied by Raicol, utilizes a monolithic bulk ppKTP cavity. A “monolithic” cavity means the reflective mirrors forming the optical resonator are coated directly onto the crystal’s end facets, creating a single, integrated device.
This innovative design dramatically boosts the effective power of a modest, off-the-shelf 1064 nm pump laser circulating inside it. The enhanced pump power then efficiently drives the difference frequency generation (DFG) process needed to convert 637 nm photons to the telecom C-band (around 1587 nm in their work), while keeping the generation of unwanted noise photons remarkably low (Fig. 1).
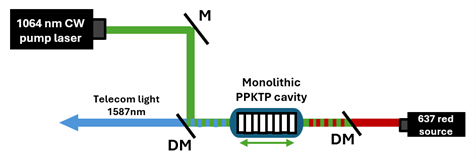
The Power of Monolithic Cavities
Efficient QFC in bulk crystals typically requires high pump powers, often necessitating expensive and complex laser systems. Resonant cavities offer a clever workaround, building up intense light fields inside the crystal from modest input powers. The monolithic design offers specific advantages: it is inherently compact and robust. Furthermore, these cavities can exhibit passive thermal self-tuning [4], meaning they can naturally stay on resonance without needing active electronic feedback loops.
This simplification significantly enhances reliability and practicality for real-world network deployment. Mann et al. [3] also hypothesize that a key advantage comes from using bulk ppKTP: the high quality of periodic poling achievable in bulk KTP [5] may intrinsically suppress parasitic nonlinear processes, particularly unwanted spontaneous parametric down-conversion (SPDC) originating from the strong pump – a major noise source in other QFC approaches.
Record Low Noise and Quantum State Preservation
The Path Forward
The Mann et al. experiment achieved an impressive internal conversion efficiency of up to 72% using only a 3 W input pump laser. Even more significantly, the noise generated was exceptionally low, measured at approximately 110 kHz/nm – a figure quantifying the rate of unwanted noise photons generated per nanometer of bandwidth at the target wavelength. This result represents a 5-fold noise reduction compared to the previous best state-of-the-art single-step converters operating at these wavelengths, dramatically increasing the feasibility of realistic quantum communication links.
Preserving the quantum nature of the light through the conversion process is crucial. The team rigorously verified this by converting single photons from an entangled pair source (SPDC). Measurements of the second-order correlation function confirmed the preservation of non-classical correlations, showing behaviour far exceeding classical limits. They also performed Franson interferometry and a chained Bell inequality test, demonstrating convincingly that time-energy entanglement was preserved with high fidelity through the conversion process.
This work highlights the significant potential of monolithic bulk ppKTP cavities as a robust, efficient, and remarkably low-noise platform for quantum network wavelength conversion. At Raicol Crystals, we provide the high-quality nonlinear crystals essential for this work, including ppKTP, apKTP, and monolithic PPKTP with broadband mirror coatings.
Our expertise in precise periodic and aperiodic poling techniques enables researchers to achieve optimal results in quantum optics applications. We also collaborate with research teams to develop customized monolithic designs that maximize efficiency while maintaining ultra-low noise profiles.
Our ongoing development of narrowband photon sources represents our commitment to advancing quantum technology. Contact Raicol Crystals to explore how our crystals can support your quantum research objectives and help bridge critical wavelength gaps in your experimental systems.
References
[1] Ruf, Maximilian, Noel H. Wan, Hyeongrak Choi, Dirk Englund, and Ronald Hanson. “Quantum networks based on color centers in diamond.” Journal of Applied Physics 130, no. 7 (2021).
[2] Dréau, Anaïs, Anna Tchebotareva, Aboubakr El Mahdaoui, Cristian Bonato, and Ronald Hanson. “Quantum frequency conversion of single photons from a nitrogen-vacancy center in diamond to telecommunication wavelengths.” Physical review applied 9, no. 6 (2018): 064031.
[3] Mann, Felix, Helen M. Chrzanowski, Felipe Gewers, Marlon Placke, and Sven Ramelow. “Low-noise quantum frequency conversion in a monolithic cavity with bulk periodically poled potassium titanyl phosphate.” Physical Review Applied 20, no. 5 (2023): 054010.
[4] Zielińska, Joanna A., and Morgan W. Mitchell. “Self-tuning optical resonator.” Optics letters 42, no. 24 (2017): 5298-5301.
[5] Mann, Felix, Helen M. Chrzanowski, and Sven Ramelow. “Low random duty-cycle errors in periodically poled KTP revealed by sum-frequency generation.” Optics Letters 46, no. 13 (2021): 3049-3052.
Have you already subscribed to our YouTube channel? Don’t miss out—subscribe now for exclusive content and updates from our company.