The Hong-Ou-Mandel (HOM) effect is a cornerstone of modern quantum optics. Originally proposed and demonstrated in 1987 by Chung-Ki Hong, Zhe-Yu Ou, and Leonard Mandel from the University of Rochester [1], the HOM effect considers a simple experiment, where two identical photons enter two ports of a beam splitter. A typical way to generate two such single photons is by using nonlinear crystals, such as PPKTP or BBO.
If one merely measures the number of photons at each output port, both ports will show on average the same counts. However, when measuring coincidence events, where photons arrive at both output ports simultaneously, one finds a quantum interference effect. We notice that there are two possible paths that result with a coincidence event: either both pass the beam splitter, or both are reflected (Fig. 1, left). Since each reflection contributes a factor of i to the phase, these two undistinguishable events have opposite phases and interfere destructively, such that no coincidence events will be measured!
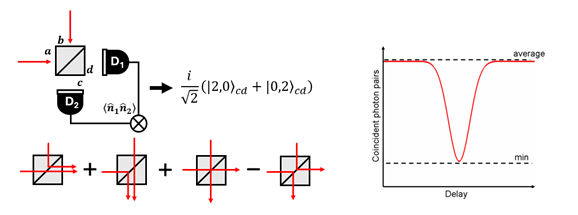
Note however, that for this interference to occur, the two photons must be completely indistinguishable. If one photon enters the beam splitter a long time after the other (longer than the coherence time of the photons), we will obviously know which photon we are measuring at each detector, and the two states will not interfere destructively. If the photons pass the beam splitter with only a slight delay between them, and their wave packets overlap (photons are not little balls! [2]), the destructive interference will occur, but with lower visibility. This is the origin of the famous “HOM dip” (Fig. 1, right), where scanning the delay between the two incoming photons results in a dip in the output coincidence counts.
In fact, in the original HOM paper, this effect was suggested as a means to measure sub-picosecond delays between the two photons, and by implication the length of the photon wave packet, with very high accuracy. Since then, the HOM effect has been used in many different contexts [3], and in particular for characterizing single photon sources such as SPDC [4] or quantum dots [5]: the depth of the HOM dip is a measure of the distinguishability between two single photons, while the width of the dip reflects the photons’ coherence length.
Recently, the HOM effect has been utilized in the context of imaging, where the HOM interference is used to reconstruct the surface depth profile of transparent samples [6]. Identical photons generated using nonlinear crystals and SPDC enter a beam-splitter, where one of the photons first passes through a sample. The different thickness of different areas of the sample induces different delays between the two photons entering the beam-splitter. As discussed above, this will result in a different number of coincidence counts, due to the HOM effect. Thus, the coincidence counts in different pixels of the detectors will reflect the corresponding thickness profile of the sample!
Compared with approaches using classical interference, HOM interference does not require phase stability of the setup, yet it can achieve similar thickness-resolution sensitivity of 1–10 nm.
Other quantum imaging modalities using single and entangled photons, N00N states, or squeezed light provide further advantages, such as sub-shot-noise measurements, better phase sensitivity, and enhanced resolution [7-9].
Besides nonlinear crystals, Raicol is currently developing entangled photon sources, which show excellent HOM visibility, and offer a complete solution for indistinguishable photons. Please contact us for more information!
[1] Hong, Chong-Ki, Zhe-Yu Ou, and Leonard Mandel. “Measurement of subpicosecond time intervals between two photons by interference.” Physical review letters 59.18 (1987): 2044.
[2] Lamb, Willis E. “Anti-photon.” Applied Physics B 60 (1995): 77-84.
[3] Bouchard, Frédéric, Alicia Sit, Yingwen Zhang, Robert Fickler, Filippo M. Miatto, Yuan Yao, Fabio Sciarrino, and Ebrahim Karimi. “Two-photon interference: the Hong–Ou–Mandel effect.” Reports on Progress in Physics 84, no. 1 (2020): 012402.
[4] Kaltenbaek, Rainer, Bibiane Blauensteiner, Marek Żukowski, Markus Aspelmeyer, and Anton Zeilinger. “Experimental interference of independent photons.” Physical review letters 96, no. 24 (2006): 240502.
[5] Sanaka, Kaoru, Alexander Pawlis, Thaddeus D. Ladd, Klaus Lischka, and Yoshihisa Yamamoto. “Indistinguishable photons from independent semiconductor nanostructures.” Physical Review Letters 103, no. 5 (2009): 053601.
[6] Ndagano, Bienvenu, Hugo Defienne, Dominic Branford, Yash D. Shah, Ashley Lyons, Niclas Westerberg, Erik M. Gauger, and Daniele Faccio. “Quantum microscopy based on Hong–Ou–Mandel interference.” Nature Photonics 16, no. 5 (2022): 384-389.
[7] Dowling, Jonathan P., and Kaushik P. Seshadreesan. “Quantum optical technologies for metrology, sensing, and imaging.” Journal of Lightwave Technology 33, no. 12 (2015): 2359-2370.
[8] Moreau, Paul-Antoine, Ermes Toninelli, Thomas Gregory, and Miles J. Padgett. “Imaging with quantum states of light.” Nature Reviews Physics 1, no. 6 (2019): 367-380.
[9] Bowen, W. P., Helen M. Chrzanowski, Dan Oron, Sven Ramelow, Dmitry Tabakaev, Alex Terrasson, and Rob Thew. “Quantum light microscopy.” Contemporary Physics (2023): 1-25.
Have you already subscribed to our YouTube channel? Don’t miss out—subscribe now for exclusive content and updates from our company.
Author: Dr. Noa Bloch
Having high threshold damage, LBO crystals for optical parametric amplification (OPA) combined with chirp pulse amplification (CPA)- (OPCPA) serves as a powerful tool to achieve very high laser peak powers (Terawatt, Petawatt). How is it done? And why LBO is the most suitable crystal for this task?
Intense light beams
Ever since lasers were invented 60 years ago, researchers have endeavored to create more intense pulses. There are many implications for intense light pulses in the industry from high precision removal material to ultrafast pulses for surgical procedures and rocket targeting in the military, but strong pulses can also unfold some fundamental questions of the universe. The holy grail of laser physics is a strong laser beam capable of breaking the vacuum of space-time. To this date, we are still a few orders of magnitude away from achieving vacuum decay. The beam intensity grew extensively over the years, however, by the mid-1980s, scientists stood upon a major challenge around 1 Terawatt of energy density. For short pulses, it was no longer practically possible to increase the intensity of the light without destroying the amplifying material.
Overcoming saturation for increasing pulse energies by chirp pulse amplification (CPA)
One method for overcoming such energy density limitations would be the increase of the laser amplifier aperture commensurate with the increase of peak power, but the required apertures would be impractically large, laser systems would be highly inefficient as they would not operate near saturation fluence, and would suffer from problems such as transverse parasitic lasing. In December 1985 the physicist Donna Strickland and her supervisor Gérard Mourou had an elegant and simple breakthrough to solve this challenge, inspired by popular science articles on radars. Their invention is simple and easy to describe – take a short laser pulse, stretch it in time, amplify it and squeeze it together again. When a pulse is stretched in time, its peak power is much lower so it can be hugely amplified without damaging the amplifier. The pulse is then compressed in time, which means that more light is packed together within a tiny area of space – and the intensity of the pulse then increases dramatically.
It took a few years for Strickland and Mourou to combine everything successfully but they overcome the technical difficulties. In 1985, Strickland and Mourou were able to prove for the first time that their elegant vision also worked in practice. Their invention, the chirped-pulse amplifier (CPA), increased intense lasers’ energy threshold up to 1 petawatt of energy density.
Combining LBO optical parametric amplifier with CPA – (OPCPA).
A further jump of one order of magnitude came later through a combination of CPA with optical parametric amplifier (OPA), known as OPCPA. The capability of delivering multi-terawatt power from a staged OPCPA system is due to additional nonlinear crystals. The most favorite for this task is LiB3O5(LBO), an attractive nonlinear crystal that can support a high efficienct and broadband second and third harmonic generation and high efficient OPCPA. Its recent development for larger size growth makes it possible for high-energy amplification. These factors indicate that a high-peak power laser system can be produced by combining a CPA and LBO booster amplifier. This nearly instantaneous nonlinear process transfers part of the energy from the pump pulse to the seed pulse, creating three outputs: a much stronger seed pulse, or signal pulse; a weakened pump pulse; and a smeared-out idler pulse at the difference frequency between the pump and the seed. A hybrid system with a CPA front end and an OPCPA final amplifier is a potential design for building a compact 10 PW laser. Thanks to LBO crystals, OPCPA has rapidly bridged the gap from its initial modest demonstrations to multi-terawatt and petawatt scale systems in research facilities and universities and numerous lower-power scientific and industrial applications.
Raicols solutins for OPCPA
Recognaising the industry need, Raicol engineered its crystal to deliver the best performance for OPCPA, offering large aperture LBOs. The crystals’ superior surface quality and optical homogeneity contribute to the lowest bulk absorption rates, which is a significant advantage for the nonlinear conversion of high-power lasers. Moreover, the unmatched surface absorption rates of Raicol’s LBO crystals ensure the highest laser damage threshold, making them ideal for high-power laser applications.
Do you have a question? Our experts will be happy to hear from you and advise you on the best product for you. Contact Us.
Have you already subscribed to our YouTube channel? Don’t miss out—subscribe now for exclusive content and updates from our company.
Author: Yoad Michael
Photonics plays a significant role in the emerging quantum information industry, and entangled photon pairs are the workhorse of many key applications in quantum optics, where each application utilizes a certain property of the quantum state.
The development of new effective sources of entangled photon pairs (EPS) is crucial for practical applications in the ever-growing field of quantum technologies, spanning from fundamental research in quantum information science, quantum communications, cryptography, sensing, imaging, and more.
There are several types of entangled photon sources which are based on different quantum states and one important source is based on polarization-entangled photon pairs, which are composed of a superposition of perpendicularly polarized signal and idler.
Unlike squeezed light, which is in principle achieved by a single-pass in a nonlinear gain medium (such as a PPKTP Crystal), polarization-entanglement requires the addition and subtraction of photons, for example by passing through more than one crystal. A recent review paper by the group of Alexander Ling [1] surveys the evolution of sources of polarization-entangled photon pairs throughout the years, as well as their possible applications in quantum communications and cryptography.
Many of the different sources shown in this review paper rely on the PPKTP Crystals that we produce at Raicol – mainly type-0 and type-II phase-matched crystals. However, the sources differ from one another in the way the crystal is utilized.
For example, in a recent quantum cryptography experiment by the University of Science and Technology of China (USTC) [2], a polarization-entangled photon source, based on type-II PPKTP Crystal placed inside a Sagnac interferometer, was used to demonstrate quantum cryptography over a distance of 1,120km. Similarly, a different bell state can also be generated using the same Sagnac configuration, with a type-0 crystal placed instead of the type-II.
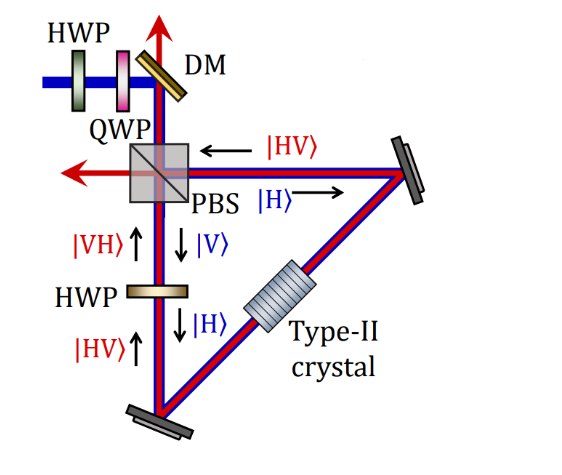
A different approach for a source of polarization entanglement, utilized by the group of Ling [3], is birefringent splitting and recombination of photon pairs. In this scheme, photon pairs are generated from two spatially-separated pump beams (where one pair experiences polarization rotation), and the beams are recombined using a birefringent material. Compensation crystals are also used to match the temporal delay between the perpendicular pairs.
![Birefringent splitting and recombination of beams, adapted from Ref [1].](https://raicol.com/wp-content/uploads/2024/03/Birefringent-splitting.png)
A third approach, which is currently being explored in Raicol, is the generation of polarization entanglement through domain engineering of the nonlinear crystal, as was shown by Kuo [4]. In this work, the researchers have designed a nonlinear crystal that can perform two simultaneous type-II processes, and showed the polarization-dependent coincidence between the two photons.
![Domain-engineered source of orthogonal pairs, adapted from Ref [1].](https://raicol.com/wp-content/uploads/2024/03/Domain-engineered-source-.png)
All of these novel methods are part of a general effort to improve sources of polarization-entangled photons, which were initially demonstrated at the intersection of two polarization cones in a type-II BBO Crystal [5]. This process relies on a non-colinear interaction, therefore rendering only a small portion of the down-converted light to be used.
As the world leader in Quasi-phase matching solutions, Raicol Quantum is proud to be part of the next quantum revolution with our advanced periodically polled crystals. For more information: www.raicol-quantum.com
Bibliography
[1] A. Anwar et al. “Entangled photon-pair sources based on three-wave mixing in bulk crystals”, Review of Scientific Instruments 92, 041101 (2021).
[2] J. Yin et al. “Entanglement-based secure quantum cryptography over 1,120 kilometres”, Nature 582, 501-505 (2020).
[3] A. Lohrmann et al. “Broadband pumped polarization entangled photon-pair source in a linear beam displacement interferometer”, Appl. Phys. Lett. 116, 021101 (2020).
[4] P.S Kuo et al. “Demonstration of a polarization-entangled photon-pair source based on phase-modulated PPLN”, OSA Continuum 3, 2, 295-304 (2020).
[5] P.G Kwiat et al. “New High-Intensity Source of Polarization-Entangled Photon Pairs”, Phys. Rev. Lett. 75, 4337 (1995).
Do you have a question? Our experts will be happy to hear from you and advise you on the best product for you. Contact Us.
Have you already subscribed to our YouTube channel? Don’t miss out—subscribe now for exclusive content and updates from our company